
The goal is to achieve a uniform speed over the intake cross section and not to waste any of the compressed air to the flow around the intake. This is done by a moveable contour of the upper intake geometry and/or the lip. The design goal is to position this cascade of shocks caused by the wedge on top such that they hit the lower intake lip. Gradually increasing the angle of the wedge is causing a cascade of ever steeper, oblique shocks which gradually decelerate the air. The picture below shows the intake of the supersonic Concorde airliner: This is done by a sequence of weak, oblique shocks and by means of a wedge intake. If the design airspeed is higher, more complex and heavier intakes are needed to decelerate the air efficiently. As a rule of thumb, a pitot intake is the best compromise at speeds below Mach 1.6. Pitot intakes with their single, straight shocks work well at low supersonic speeds, but incur higher losses at higher Mach numbers. Weak shocks are desired, because they produce only small losses due to friction. The angle of the oblique shock wave is determined by the Mach number ahead of the shock. Now the speed $v_2$ is still supersonic, but lower than $v_1$, so a weak oblique shock produces a modest increase of pressure, density and temperature.
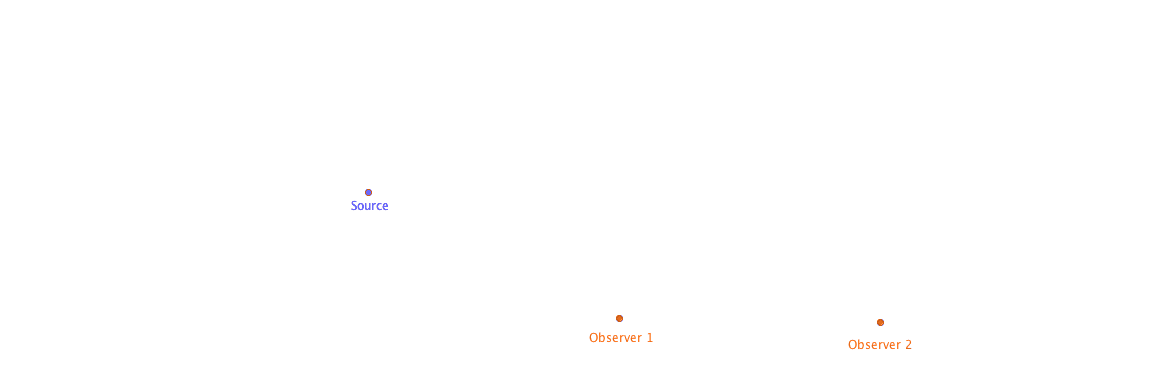
Note that the tangential component $v_t$ is unaffected by the shock! Only the normal component is reduced. The equation for the stagnation temperature $T_0$ of air shows how important the flight speed $v$ is: $$T_0 = T_ = a^2$$ Of course, even a 6,000° K flame temperature is a challenge for the materials of the combustion chamber, and ceramics with film cooling are mandatory. The 6,000° K figure above is a rough compromise for the boundary where adding more energy starts to make less and less sense. Mach 2 to still achieve combustion with a meaningful temperature increase - that is why scramjets are used in hypersonic vehicles.įull disclosure: Oxygen starts to dissociate already between 2,000° and 4,000° K, depending on pressure, while Nitrogen will dissociate mainly above 8,000° K. If the air enters the intake at Mach 6, it must not be decelerated below approx. Since thrust is produced by expanding air through heating, burning air that enters the combustion process already at 6,000° K will not achieve much thrust. 6,000° K, adding more energy will result in dissociation of the gas with little further heat increase. This compression heats the air, and in order to achieve a combustion which produces thrust, this heating must be restricted. Due to the high flight speeds, compression is possible by a cascade of shocks, so no moving turbomachinery is needed in ramjets and scramjets.Īll jets decelerate air in their intake in order to increase air pressure. By using hydrogen, a stable combustion can be achieved even in supersonic flow. If the engine would burn regular kerosene, the flame would be blown out like a candle if the internal airspeed would be supersonic, and even if flame holders keep the flame in place, most combustion would take place only after the fuel-air mixture has left the engine due to the slow mixing of kerosene and air. Note that the high circumferential speed of a large fan blade will still mean that its tips work at around Mach 1.5, but the subsequent compressor stages will operate in subsonic conditions.Ī scramjet is possible with fuels with supersonic flame front speeds and rapid mixing of fuel and air. Thus, the intake has to slow down the air to a Mach number between 0.4 and 0.5. Supersonic flow introduces additional drag sources which should be avoided if efficiency is important.
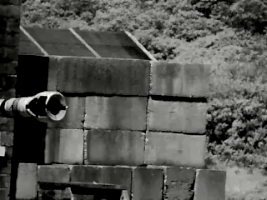
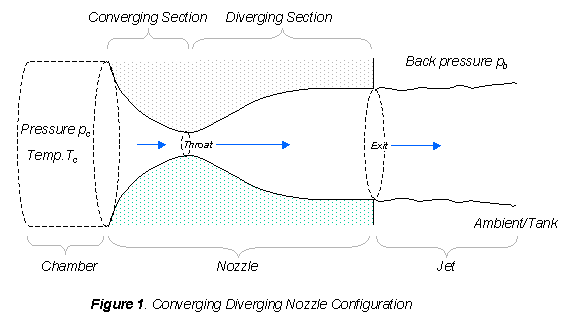
A compressor blade works best in subsonic flow.
